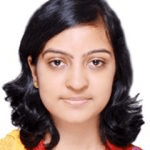
Unnati Ashar
Scientists in the Tata Institute of Fundamental Research (TIFR), Mumbai, Vikram Sarabhai Space Centre, Trivandrum, and Inter-University Centre for Astronomy and Astrophysics (IUCAA), Pune, developed the Cadmium Zinc Telluride Imager, one of the instruments onboard AstroSat. In addition to scientists from IUCAA and TIFR, scientists from the Physical Research Laboratory (PRL), Ahmedabad and the Indian Institute of Technology (IIT)- Bombay, study the data collected over the last six years of its operation. They refer to it as the CZTI in short.
The quanta of light are photons. In the X-ray wavelengths, astronomers find it easier to express the photons’ energies with a unit they call the ‘kiloelectronvolt’. An X-ray photon with an energy of one keV has a wavelength of about a nanometre, ten thousand times smaller than the human hair. The X-ray photons that bombard the CZTI have energy in the range of about 10 to about 150 kiloelectronvolts. Their wavelengths are similar to the radii of atoms.
Telescopes that detect light of visible wavelengths, or ‘optical telescopes’, use lenses and mirrors to focus visible light. Having much shorter wavelengths than visible light, X-rays shoot through the mirrors and lenses of these telescopes. Hence, to focus the X-rays, the CZTI’s scientists devised different techniques.
A ‘coded aperture mask’ is one such tool. It is a grid of patterns that is partially opaque to X-ray and gamma-ray photons and partially allows them to pass through. The scientists find the properties of the astronomical source of these photons by studying the shadow that it casts on a plane situated below the mask. The plane contains pixels of detectors, just like a modern camera. The detectors, made of Cadmium Zinc Telluride, respond to the incoming X-ray photons. To decipher the properties of the astronomical source, scientists use algorithms to process the data from the pixelated detectors. A type of coded mask designed by Minoru Oda opened up the field of X-ray astronomy by detecting the first cosmic X-ray source.
Before a satellite is launched, and even after it’s in orbit, the instruments on the satellite have to undergo a process that scientists call ‘calibration’. Calibrating an astronomy instrument like the CZTI means understanding how the pixels behave and what shadow the aperture mask casts when the astronomical sources are in different directions. For this, scientists use astronomical sources whose properties are well-known from decades of study. Once they have calibrated an instrument, they can measure the unknown properties of any astronomical source.
The CZTI scientists calibrated the instrument both before and after sending it to space. In the laboratory, they exposed the CZTI to a radioactive source placed in front of it. In space, they calibrated it by pointing it at well-known astronomical sources. Then, they used data processing algorithms to construct the image of unknown sources and compared it with expected images from previous knowledge.
The scientists found that either the constructed image was not located exactly where they expected it or was elongated. Further investigating the data, they determined that the coded aperture mask and the detector were not perfectly aligned. Then, they tweaked the data processing algorithms and corrected the anomaly.
The CZTI contains a collimator of height 40 centimetres between the coded mask and the pixelated detectors, limiting the area in the sky from which photons reach the detector plane. However, photons of 100 kiloelectronvolt easily pass through the coded mask and the collimator. Hence, photons greater than 100 kiloelectronvolts can reach the pixelated detectors from all directions in the sky. Thus, while the four other instruments on AstroSat are busy observing specific astronomical sources, CZTI observes a large area of the sky.
Some astronomical sources emit a burst of X-ray photons for a few seconds before disappearing forever, which astronomers call ‘transient’ sources. The CZTI can detect transient sources that emit photons of greater than 100 kiloelectronvolt energies from all parts of the sky. And though this is a useful capability, to detect more information about the transients, astronomers need to know from which direction the X-ray photons of the transient are arriving.
By the time the X-ray photons reach the CZTI, they interact with various parts of the satellite and undergo absorption, scattering, reflection, and re-emission in different directions. For scientists, understanding how the various elements of the satellite interact with the X-rays is crucial to determining the properties of the astronomical sources of these photons.
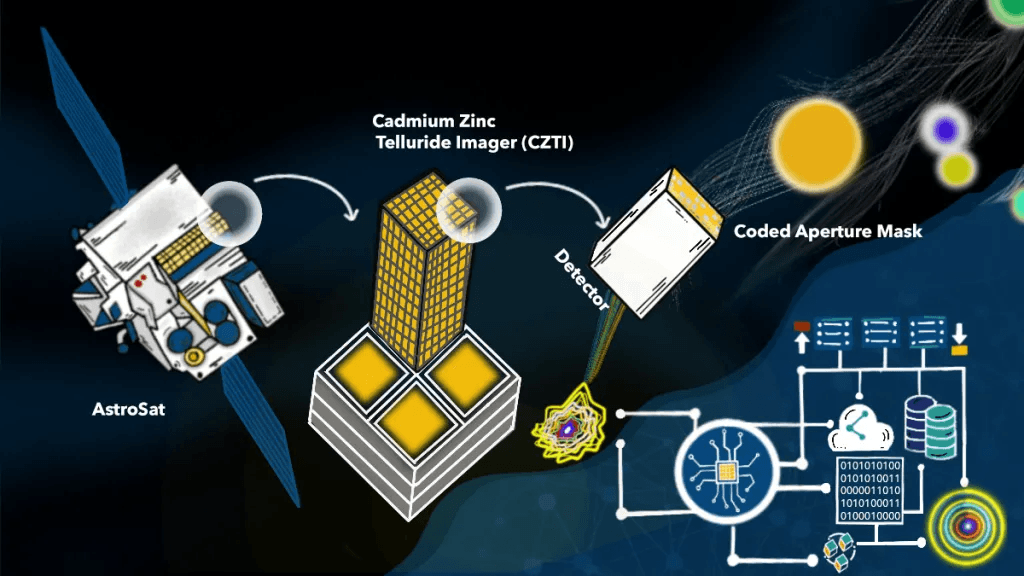
A graphical representation of the workings of CZTI.
Illustrated by: Rupsy Khurana
The CZTI scientists created a model of the distribution of AstroSat’s mass. In the model, they simulated how the satellite’s mass interacts with incoming X-ray energy photons. As different elements of the satellite body cast shadows on the CZTI’s detector plane, scientists can infer the direction of the sky from which the X-ray photons bombarded the detector plane by comparing the observed data with their simulations.
For validating the model, the CZTI scientists compared observations of transient sources with the model simulations. While the model’s predictions matched the observed CZTI data for a few sources, for others, they found significant discrepancies between the data and the predictions. Scientists attribute the discrepancies to missing components in their model. However, questions to the scientists for clarification and present and future ways of improving the model remained unanswered at the time of writing this series.
In the following article in the series, “An AstroSat instrument is scanning the sky with X-rays“, we’ll see how scientists extended the range of wavelengths in which the Cadmium Zinc Telluride Imager operates.